By Misbah A. Moten, Asma S. Ansari, Farzeen S. Waseem, Ume Hani
AFFLIATIONS:
Department of Oral Biology, Dr. Ishrat-ul-Ebad Khan Institute of Oral Health Sciences, Dow University of Health Sciences, Karachi, Pakistan.
DOI: https://doi.org/10.36283/PJMD11-3/011
ORCID iD: 0000-0002-4526-0530
How to cite: Moten MA, Ansari AS, Waseem FS, Hani U. Alveolar Bone Regeneration Via Utilization of Nanohydroxyapatite Scaffolds: A Review. Pak J Med Dent. 2022;11(3): 66-74. doi: 10.36283/PJMD11-3/011
The complete architectural and functional rehabilitation of periodontium owes to the integrity of alveolar bone. The inherent shortcomings of traditional gold standard regenerative procedures like autografting, xenografting, allografting and alloplasting lead to the evolutionary combination of Tissue Engineering/Regenerative Medicine (TE/RM) and nanotechnology for bone repair. Nanotechnology enables the fabrication of either nanoparticles, nanofibers or nanocomposites based on three-dimensional scaffolds. However, it will incorporate vital cells and growth factors in various combinations, to simulate a conducive oral environment of the extracellular matrix (ECM) and empower cells in the bone to regulate in-vivo osteogenesis. The advantageous combination of structural similarity of nanohydroxyapatite (nHA) Scaffolds to the alveolar bone with favorable particle size, response rate, tissue factors and bio factor, makes it attractive for TE/RM. Relevant keywords from 2010-2021 studies were used to retrieve data from “PubMed” and “Google Scholar”. This review aims to summarize the cumulative knowledge of commercially available nanohydroxyapatite scaffolds for utilization in alveolar bone augmentation, regeneration of implant osteointegration by their fabrication techniques, advantages, components, types, interaction with various components and particular application of each type for in vivo alveolar bone regeneration. Therefore, nHA scaffolds possess significant osteoconductive and osteoinductive effects on structural similarities to the composition, adhesion and differentiation of bone-forming cells.
Keywords: Scaffolds; Tissue Engineering; Nano-Hydroxyapatite, Bone Regeneration; Nanotechnology.
To achieve complete restoration of tissue function, following defects or diseases, by regeneration1, requires biocompatible bone substitute materials2-4. Xenografting and allografting are associated with high infection potential, immune rejection, and lower revascularization. The utilization of the multidisciplinary field of TE/RM, having biocompatible materials imitating the natural environment of ECM5,6 have drawbacks of insufficient strength and physicochemical properties2. This led to the exploration of further materials2,3,7. The articles reviewed were selected by searching keywords such as “Nanohydroxyapatite scaffolds”, “alveolar bone regeneration”, “nano scaffolds”, “nanomaterial” and “nanotechnology” from the databases of “PubMed” and “Google Scholar”. All articles are within 10 years limit of this review without limitations on study design. Previously published reviews were also included. This review will also focus on the application of a nano scaffold component of TE/RM based on available nanohydroxyapatite scaffolds for in vivo alveolar bone regeneration. The objective of the review is to summarize the current pool of knowledge on commercially available nanohydroxyapatite for the clinicians dealing with alveolar bone augmentation, regeneration, or reinforcement of implant osteointegration according to their fabrication techniques. The types, their interaction with various components, their advantages and application of each type related to in vivo alveolar bone regeneration are also highlighted.
A nanomaterial can be defined as: ‘A natural, incidental or manufactured material containing particles, in an unbound state or as an aggregate and where, for 50% or more of the particles in the number size distribution, one or more external dimensions is in the size range of 1 nm–100 nm 8. Nanomaterials can either have one, two or all dimensions <100 nm and are known as nanocomposites, nanofibers or nanoparticles respectively9.
Nano scaffolds have been fabricated for many of the limitations of the conventional scaffolds by its likeness to the tissue-specific ECM; the enhanced speed of response to stimuli such as ultrasounds, pH and other stimuli; controlled release of bio factors, drug and genetic materials using their smaller particle size and high tissue specificity; increased stability of bioactive agents; elaborated drug loading capability with enhanced mobility of such particles and good reactivity for tissues2.
Nano-fibrous Scaffolds
Highly porous and mechanically strong structures with unremitting very small diameter fibers provide a diffusional path with a considerable surface area/mass ratio. Excellent transporting medium by about drug delivery as well2.
Nanosphere Scaffolds
These porous structures (porosity increased usually after the addition of spheres like porogen) although mechanically weak, strength is gained by cross-linking nanospheres as an adjunct are useful for delivery of growth factors, drugs, or genetic material. They also provide useful self-hardening biomaterials for bone tissue regeneration since they stimulate the formation of apatite crystals after the mineralization of hydrogels2. The remarkable similarity in composition of nanomaterial and alveolar bone has enabled its use for alveolar bone regenerating procedures.
Organic Phase: Arrangement of type I collagen into 50 to 500 nm nanofibers.
Inorganic Phase: Embedded between collagen fibers are about 100 nm long, 20-30 nm wide and 3-6 nm thick non-stoichiometric hydroxyapatite (HA)10. Because of this resemblance to the inherent composition of bone, nanohydroxyapatite (nHA) scaffolds have gained great interest in the field of TE/RM11. Hence, In the field of oral biology, covering areas of regeneration, nanotechnology plays a vital role2.
Advantages of Combined Bone Nano Scaffolds with Hydroxyapatite
The naturally occurring mineral Hydroxyapatite [Hap Ca10(OH)2(PO4)6] in almost all dental hard issues contributes many advantages solely by its presence in the bone constructs12,13. Its compositional resemblance to the tissues grants sufficient biocompatibility along with proven osteoconductive and osteoinductive properties13. Furthermore, its porous nature makes it a house for capillaries and cells and leading to perfusion and delivery of metabolic oxygen to cells present within scaffolds and host cells nearby. It shows slow biodegradability and provides an enhancement of surface properties of scaffolds which could improve fracture toughness and charge, making it able to change adsorbed biomolecules4. It promotes cell response and cell proliferation needed for bone TE/RM14.
Advantages of Nano Scaffold Systems
Nano-scaffold systems provide mass transport due to the porous structure being large and interconnected, having the ability to bear shear stresses during the cultivation of bioreactor because of its stable structure. Its hydrophilic nature provides an enhancement of cell attachment and biocompatibility due to its biodegradable nature15,16. Lastly, the elastic property of nano scaffolds accounts for the transmission of contractile forces.
Components of Nanohydroxyapatite Scaffolds Favoring Alveolar Bone Regeneration
Although the advantages of bone constructs through hydroxyapatite alone are priceless, their limited mechanical strength, slow degradation rates, brittleness and fatigue failure, necessitate the enhancement of their osteoconductive and osteoinductive properties, substituting for conventional bone grafts, they occur as polymers and bioceramics such as hydroxyapatite [HAp, Ca10(OH)2(PO4) 6] incorporated composites17. This polymer/bio ceramic composites combination promotes osteogenic differentiation, mineralization and high affinity for adhesive proteins18. Incorporation of polymers and bioceramics can occur either by integration of compounds within polymeric matrices or surface coating of bio-ceramic on the surface of scaffolds for improved properties (Figure 1). The latter concept is more commonly employed18.
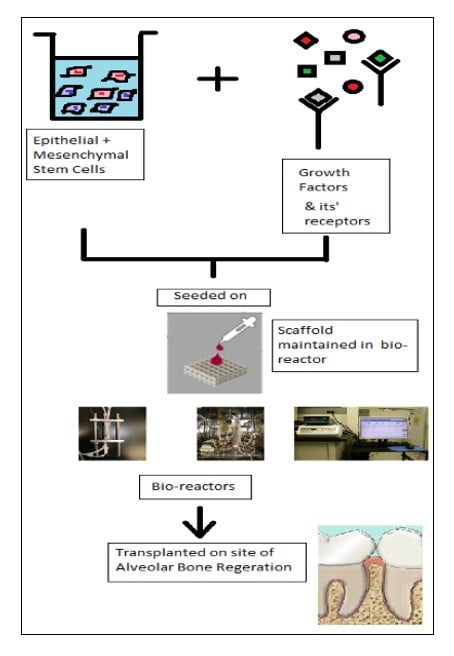
Figure 1: Diagrammatic representation of scaffold constructs for regeneration of tooth and its supporting tissues demonstrating seeding of growth factors and epithelial and mesenchymal cells on composite scaffolds.
Polymers
Polymers occurring in combination with hydroxyapatite nano scaffolds (Table 1):
Table 1: Polymers occurring in combination with hydroxyapatite nano scaffolds.
Natural Polymers | Synthetic Polymers |
Chitosan (CTS) | Poly-Lactic-Glycolic Acid (PLGA) |
Collagen (Col) | Poly-Caprolactone (PCL) |
Coralline | Poly-Α-Hydroxyesters (Poly-Glycolic Acid (PGA) |
Alginate | Poly (L-Lactic Acid) |
Gelatin | Poly-Lactic Acid (PLA) |
PLLA/Polyethylene Glycol (PEG) | |
Dendrimer | |
Silk fibroin | |
Polyurethane |
Natural Polymers: Chitosan (CTS)
Nanohydroxyapatite-chitosan scaffolds have favorable outcomes in terms of improvement in mechanical properties and bio-mineralization of either natural chitosan-based systems, lack bioactivity to achieve hard tissue or when HAp-based systems are used alone19. In vitro studies have shown the positive outcome of alveolar bone-like structure regeneration and improvement in osteogenic differentiation by use of PDLSCs20. Moreover, hydroxypropyl methylcellulose (HPMC) incorporated Chitosan/HA scaffolds also have potential in terms of alveolar bone regeneration21.
Collagen (COL)
The combination of bone marrow-derived stem cells (hBMSCS) and collagen with nanohydroxyapatite can stimulate osteogenic differentiation after 7 days utilizing the 3D printing method22. The nHA/Col scaffolds demonstrated significant signs of osteogenic differentiation of mouse calvarial MC3T3-E1 osteoblasts precursor cells line along with natural bone ceramic/collagen scaffolds when compared in a study23. Also interestingly, increased areas of mineralization were found in naturally occurring collagen HA scaffolds versus commercial scaffolds24.
Coralline
In combination with HA, coralline for nHA/coral scaffolds as CHA particles have demonstrated increased periodontal ligament cell attachment initially in case of regeneration of periodontium25.
Synthetic Polymers
These are hydrophilic and lack cell affinity and bioactivity24,26.
PLGA/PLLA/PEG/PCL
These four synthetic polymers PLGA/PLLA/PEG/PCL in different combinations have been shown to produce alveolar bone regeneration2.
Bioceramics Component of Nanohydroxyapatite Bone Scaffolds: Ceramic Composite Scaffolds
Added to scaffolds for better performance in terms of delivery of materials and to improve structural properties2. They include Mineral Trioxide Aggregate (MTA), CP Groups (Calcium Phosphate Groups) and Bioactive Glass Ceramic (Na2O–CaO–SiO2–P2O5) 27.
Bioactive Glass Ceramic
Nano scaffolds containing bioactive glass have shown to be advantageous for new bone regeneration28.
CP Groups (Calcium Phosphate Groups)
The most frequently used materials for tissue regeneration are CP nanoparticles (nano-tricalcium phosphate (nTCP) and nHA in various combinations due to structural similarities with bone29. A combination of nHA with chitosan, polyamide, collagen, PLGA, PLA, coralline and PCL has demonstrated beneficial improved mechanical strength and biocompatibility2. Since collagen offers an osteoinductive effect and helps in producing absorbable scaffolds for osteoblasts incorporation so in PCL composite materials percentages of nHA and collagen are carefully controlled for favorable properties as nHA being nonabsorbable can lessen the effect of collagen fibers30.
Methods of Preparation of Nanohydroxyapatite Scaffolds – Solvent-Solution Casting Methods: It requires minimal instrumentation or specialized techniques and carries the advantageous control over pore size, employing dissolving the polymers into organic solvents with the subsequent addition of ceramic granules, followed by casting into 3D molds and subsequent evaporation of organic solvent31,32. PLGA/nHA scaffolds with sizes 50-200µm have been successfully produced by a combination of solvent solution casting and freeze dying32. The toxicity of solvents limits the use of this technique33.
Freeze Dying Method Or Thermally Induced Phase Separation (TIPS): It represents a promising technique for nano-fibers scaffolds, involves separation into polymer enriched and polymer poor phases with subsequent freeze-drying, of homogenous solution of the polymer at specific conditions34, owing to efficacy of solvent decreases proportionally with decreasing temperature35. It gives good control of pore size and shape36 and has shown limitations of inadequate mechanical properties, poor fiber orientation and size and time consumption37. PLLA/nHA have been fabricated using TIPS providing combined benefits of both components with advantages of the technique38. Novel porous scaffolds such as nHA/Col/PLLA/CTS microsphere have also been produced using TIPS39. Nano scaffolds capable of controlled release of BMP-2 have been produced by this method as well40. The use of the freeze-dying method has also allowed the fabrication of a novel nHA/CTS/CMC which has popular use as a potential substitute for TE/RM as it has demonstrated good bioactivity and biodegradability in in-vitro tests41.
Electro Spinning: The versatile and revolutionary method of producing polymer-based high-quality nano-fibers42, is a frequently used technique and one of the most promising scaffold-fabricating technique43. It includes advantages of ultra-thin film deposition of biomaterials both organic and inorganic due to increased surface area, bioactive material delivery, safety and greater productivity44-46. The electric field deposits the material as the surface tension of the melt (polymers) is surmounted by the pulling nature of the electric field, leading to evaporation of the solvent47. Nano scaffolds using both natural polymers such as silk fibroin, chitosan (CTS), collagen (Col) and gelatin and synthetic polymers such as PLGA, PLLA, polyurethane, PGA, PCL and their combinations have been successfully prepared by electrospinning48. Novel nHA/CTS nanofibrous scaffolds using combined co-precipitation and electrospinning have demonstrated better results in terms of bone formation as compared to electrospinning alone49. The scaffolds of nHA/Gelatin, nHA/PLA, triphasic nHA/Col/PCL and silk/nHA produced previously with electrospinning of blended inorganic nanoparticles with viscous solutions resulted in limitations of scaffolds incapable of interacting between organic and inorganic phases50,51.
Self-Assembly
components are organized into patterns or structures without man force utilizing hydrophilic interactions, non-covalent bonding, electrostatic and Vander Walls forces of interaction52. It is the most cost-effective method and does not possess the difficulty of involvement of many steps and modification53. Reduced Graphene Oxide (RGO) together with nanohydroxyapatite has also been used to construct a porous nano-scaffold by this technique and offers favorable bone tissue repair54.
Salt Leaching
This technique has been used to generate porous polymers such as polylactide/nHA and poly (hydroxybutyrate-co- hydroxyvalerate) PHBV/ polycaprolactone PCL/nHA offering success as scaffolds for bone tissue engineering55,56.
3D Printing
This technique paves the way to overcoming limitations of porosity factors linked with traditional techniques including poor control over pore size and its interconnectivity by interactions between bioactive cells and hydrogels for facilitation of tissue regeneration making use of a CAD program57.
Other Techniques
Nano-hydroxyapatite scaffolds have also been prepared using other techniques including meltdown, template synthesis, gas foaming and sponge replication56,58,59.
Applications of Nanohydroxyapatite Scaffolds for Alveolar Bone Regeneration
Studies testing nanohydroxyapatite scaffold application concerning alveolar bone regeneration are shown in Table 2.
Table 2: Studies testing nanohydroxyapatite scaffold applications.
Author(s) | Year | Scaffold Material | Concluding Remarks |
Neto et al. 60 | 2018 | Nano-hydroxyapatite
coral scaffolds CHA |
Pre-vascularized nHA/coral
potentially significant alveolar bone regeneration |
Wang et al. 61 | 2018 | Insulin loaded
nHA/Col/PLGA Composite scaffold |
nHAC bone regeneration
capability enhancement using such a scaffold |
Jang et al. 62 | 2017 | Porous HA scaffold | PHA superiority over HA granules in terms of neo bone formation 8 weeks post-implantation |
Wu et al. 63 | 2018 | BMP-2 mediated
DPSCs seeded nHAC/PLA |
rhBMP-2 nHAC/PLA promising for PDL bone regeneration |
Chen et al. 20 | 2016 | GelMA/ nHA) | GelMA/nHA microgels potential candidates for PDL regeneration |
Yan et al. 64 | 2018 | PLGA/nHA/CMs/ADM | Successful results in terms of deceleration of Residual Ridge Resorption and increase in remodeling of Alveolar Bone when used without ADM |
Nano-Hydroxyapatite Coral Scaffolds Coralline Hydroxyapatite (CHA)
Although, composite scaffolds such as nano-hydroxyapatite coral scaffolds CHA having an inner core of coralline and outer HA layer have demonstrated good clinical applications owing to its osteogenic effects. Nonetheless, favorable outcomes for bone regeneration have not yet been achieved as it’s dependent on neovascularization and recruiting progenitor cells at the site of damage (Table 2). Therefore, nHA/coral scaffold blocks were coated with recombinant rhVEGF165 to pre-vascularize block grafts and enhance angiogenesis, tested on critical size mandibular defects on experimental animals revealed good results in the early stages of healing. This makes pre-vascularized nHA/coral a potentially significant material for alveolar defects regeneration60.
Insulin Loaded nHA/Col/PLGA Composite Scaffold for Enhanced Bone Regeneration and Repair
To obtain the combined benefits of drug delivery attributable to PLGA, and excellent cell attachment, proliferation and porosity for scaffolds by nHA/Col and the ability of insulin to promote bone turnover and osteogenesis, a combination of these materials as a composite scaffold by Wang et al. resulted in great tissue compatibility, differentiation capacity of BMSCs to osteoblasts and bone restoration capacity, when compared to nHA/Col, used alone61.
Porous HA scaffold – Ideal for Repairing Alveolar Sockets
An in vivo study extracted canine sockets filled with either granular or porous hydroxyapatite scaffolds to compare their efficacy for alveolar bone regeneration which revealed the faster formation of new bone with porous HA scaffold than that with granular HA making it a suitable material for osteogenesis. Furthermore, isotropically porous HA scaffold allowed complete healing of alveolar socket62.
Role of BMP-2 Mediated DPSCs Seeded Nano-Hydroxyapatite/Collagen/Poly (L-lactide)
Tissue-engineered bone with the use of biodegradable nHAC/PLA scaffold mediated with rhBMP-2 and seeded with DPSCs revealed earlier achievement of mineralization and increased amount of bone formation in comparison to either use of nHAC/PLA alone or a combination of nHAC/PLA only with rhBMP-2 without DPSCs or use of nHAC/PLA + DPSCs without rhBMP-2 or even autologous bone ensuring the combination of the above stated three as a suitable material for alveolar bone defect reconstruction63.
Gelatin methacrylate/Nanohydroxyapatite Microgel Arrays (Gel MA / nHA)
In an in vitro study, great regeneration of new bone in experimental mice was suggested with the use of GelMA/nHA microgels opening doors to its evaluation as a potential material for periodontal tissue regeneration20.
Chitosan Microspheres Nano-Hydroxyapatite PLGA Scaffolds Delivering Adrenomedullin
Remodeling of Alveolar Bone and reduction in residual ridge resorption can be accelerated better with PLGA/nHA/CMs/ADM composite scaffolds as compared to PLGA/nHA/CMs scaffolds when used without ADM64.
The review of literature about experimental studies has demonstrated significant osteoconductive and osteoinductive effects of Nano-Hydroxyapatite (nHA) scaffolds by possessing structural similarities to the composition of bone, it shows excellent adhesion to bone and differentiation of bone-forming cells along with its biocompatibility. The application of various nHA composites has been reported to be useful for GTR (guided tissue regeneration). Furthermore, the achievement of angiogenetic properties for Nanohydroxyapatite scaffolds using VEGF has added to the osteogenic regeneration capacity. Also, since nHA shows short-term resorbability it’s an added advantage for the preservation of post-extraction socket and reducing remaining graft material around implants. Then HA properties make it an equally applicable alternative to autogenous grafting materials in the treatment of intrabony defects of the periodontium. Its osteoinductive potentials have been shown to improve with the coating of VEGF and other growth factors about enhanced angiogenesis. Therefore, if the biological properties and usage of Nanohydroxyapatite scaffolds must be improved, other factors should be incorporated and further randomized controlled trials are needed for future evaluation.
The authors would like to acknowledge the department and colleagues for their constant motivation and support.
The authors declared no conflict of interest.
MM designed and wrote the manuscript and is the corresponding author, AA designed the concept, drafted and critically reviewed the article, FW finalized the concept design and proofread whereas UH proofread and managed the references.
- Nanci A. Ten Cate’s Oral Histology-e-book: development, structure, and function [Internet]. Elsevier Health Sciences; 2017. 10p.
- Funda G, Taschieri S, Bruno GA, Grecchi E, Paolo S, Girolamo D, et al. Nanotechnology scaffolds for alveolar bone regeneration. Materials. 2020;13(1):1-20. doi: 10.3390/ma13010201
- Cho YS, Quan M, Kang NU, Jeong HJ, Hong MW, Kim YY, et al. Strategy for enhancing mechanical properties and bone regeneration of 3D polycaprolactone kagome scaffold: Nano hydroxyapatite composite and its exposure. Eur Polym J. 2020;134:1-12. doi: 10.1016/j.eurpolymj.2020.109814
- Zakrzewski W, Dobrzynski M, Rybak Z, Szymonowicz M, Wiglusz RJ. Selected nanomaterials’ application enhanced with the use of stem cells in acceleration of alveolar bone regeneration during augmentation process. Nanomaterials. 2020;10(6):1-29. doi: 10.3390/nano10061216
- Kubasiewicz-Ross P, Hadzik J, Seeliger J, Kozak K, Jurczyszyn K, Gerber H, et al. New nano-hydroxyapatite in bone defect regeneration: A histological study in rats. Ann Anat. 2017;213:83-90. doi: 10.1016/j.aanat.2017.05.010
- Gautam S, Sharma C, Purohit SD, Singh H, Dinda AK, Potdar PD, Chou CF, Mishra NC. Gelatin-polycaprolactone-nanohydroxyapatite electrospun nanocomposite scaffold for bone tissue engineering. M Mater Sci Eng C. 2021;119:1-14. doi: 10.1016/j.msec.2020.111588
- Titsinides S, Agrogiannis G, Karatzas T. Bone grafting materials in dentoalveolar reconstruction: A comprehensive review. Jpn Dent Sci Rev. 2019;55(1):26-32. doi: 10.1016/j.jdsr.2018.09.003
- Dorozhkin SV. Nanometric calcium orthophosphates (capo4): Preparation, properties and biomedical applications. Adv Nano Bio M&D. 2019;3:422-513.
- Jeevanandam J, Barhoum A, Chan YS, Dufresne A, Danquah MK. Review on nanoparticles and nanostructured materials: history, sources, toxicity and regulations. Beilstein J Nanotechnol. 2018;9(1):1050-1074. doi: 10.3762/bjnano.9.98
- Phatai P, Futalan CM, Utara S, Khemthong P, Kamonwannasit S. Structural characterization of cerium-doped hydroxyapatite nanoparticles synthesized by an ultrasonic-assisted sol-gel technique. Results Phys. 2018;10:956-963. doi: 10.1016/j.rinp.2018.08.012
- Anil L, Vandana KL. Three dimensional printed scaffolds and biomaterials for periodontal regeneration-an insight. Int J Mol Biol Open Access. 2020;5:73-77. doi: 10.15406/ijmboa.2020.05.00135
- Michel J, Penna M, Kochen J, Cheung H. Recent advances in hydroxyapatite scaffolds containing mesenchymal stem cells. Stem Cells Int. 2015;2015:1-13. doi: 10.1155/2015/305217
- Lim JW, Jang KJ, Son H, Park S, Kim JE, Kim HB, et al. Aligned nanofiber-guided bone regeneration barrier incorporated with equine bone-derived hydroxyapatite for alveolar bone regeneration. Polymers. 2020;13(1):1-11. doi: 10.3390/polym13010060
- Ruphuy G, Souto‐Lopes M, Paiva D, Costa P, Rodrigues AE, Monteiro FJ, et al. Supercritical co2 assisted process for the production of high‐purity and sterile nano‐hydroxyapatite/chitosan hybrid scaffolds. J Biomed Mater Res B Appl Biomater. 2018;106(3):965-975. doi : 10.1002/jbm.b.33903
- Fathi-Achachelouei M, Knopf-Marques H, Ribeiro da Silva CE, Barthès J, Bat E, Tezcaner A, et al. Use of nanoparticles in tissue engineering and regenerative medicine. Front Bioeng Biotechnol. 2019;7:1-22. doi: 10.3389/fbioe.2019.00113
- Lyons JG, Plantz MA, Hsu WK, Hsu EL, Minardi S. Nanostructured biomaterials for bone regeneration. Front Bioeng Biotechnol. 2020:1-28. doi: 10.3389/fbioe.2020.00922
- Liu J, Zou Q, Wang C, Lin M, Li Y, Zhang R, et al. Electrospinning and 3D printed hybrid bi-layer scaffold for guided bone regeneration. Mater Des. 2021;210:1-13. doi: 10.1016/j.matdes.2021.110047
- Lee JB, Kim JE, Balikov DA, Bae MS, Heo DN, Lee D, et al. Poly (l‐lactic acid)/gelatin fibrous scaffold loaded with simvastatin/beta‐cyclodextrin‐modified hydroxyapatite inclusion complex for bone tissue regeneration. Macromol Biosci. 2016;16(7):1027-1038. doi: 10.1002/mabi.201500450
- Lauritano D, Limongelli L, Moreo G, Favia G, Carinci F. Nanomaterials for periodontal tissue engineering: chitosan-based scaffolds. A systematic review. Nanomaterials. 2020;10(4):1-16. doi: 10.3390/nano10040605
- Chen X, Bai S, Li B, Liu H, Wu G, Liu S, et al. Fabrication of gelatin methacrylate/nanohydroxyapatite microgel arrays for periodontal tissue regeneration. Int J Nanomedicine. 2016; 11: 4707-4718. doi: 10.2147/IJN.S111701
- Iqbal H, Ali M, Zeeshan R, Mutahir Z, Iqbal F, Nawaz MA, et al. Chitosan/hydroxyapatite (HA)/hydroxypropylmethyl cellulose (HPMC) spongy scaffolds-synthesis and evaluation as potential alveolar bone substitutes. Colloids Surf B Biointerfaces. 2017;160:553-563. doi: 10.1016/j.colsurfb.2017.09.059
- Li Q, Lei X, Wang X, Cai Z, Lyu P, Zhang G. Hydroxyapatite/collagen three-dimensional printed scaffolds and their osteogenic effects on human bone marrow-derived mesenchymal stem cells. Tissue Engineering Part A. 2019;25(17-18):1261-1271. doi: 10.1089/ten.tea.2018.0201
- Lei X, Gao J, Xing F, Zhang Y, Ma Y, Zhang G. Comparative evaluation of the physicochemical properties of nano-hydroxyapatite/collagen and natural bone ceramic/collagen scaffolds and their osteogenesis-promoting effect on MC3T3-E1 cells. Regen Biomater. 2019;6(6):361-371. doi: 10.1093/rb/rbz026
- Griffin MF, Kalaskar DM, Seifalian A, Butler PE. Suppl-3, M4: an update on the application of nanotechnology in bone tissue engineering. Open Orthop J. 2016; 10: 836-848. doi: 10.2174/1874325001610010836
- McPherson R, Vickers PG, Slater GL. Bone grafting with coralline hydroxyapatite. EC Dent. Sci. 2019;18:2413-23.
- Du M, Chen J, Liu K, Xing H, Song C. Recent advances in biomedical engineering of nano-hydroxyapatite including dentistry, cancer treatment and bone repair. Compos B Eng. 2021;215:1-23. doi: 10.1016/j.compositesb.2021.108790
- Venkatesan J, Kim SK. Nano-hydroxyapatite composite biomaterials for bone tissue engineering—a review. J Biomed Nanotechnol. 2014;10(10):3124-3140. doi: 10.1166/jbn.2014.1893
- Zhang J, Chen Y, Xu J, Wang J, Li C, Wang L. Tissue engineering using 3D printed nano-bioactive glass loaded with NELL1 gene for repairing alveolar bone defects. Regen Biomater. 2018;5(4):213-220. doi: 10.1093/rb/rby015
- Manju V, Anitha A, Menon D, Iyer S, Nair SV, Nair MB. Nanofibrous yarn reinforced HA-gelatin composite scaffolds promote bone formation in critical sized alveolar defects in rabbit model. Biomed Mater. 2018;13(6):1-31. doi: 10.1088/1748-605X/aadf99
- Wang X, Xing H, Zhang G, Wu X, Zou X, Feng L, et al. Restoration of a critical mandibular bone defect using human alveolar bone-derived stem cells and porous nano-HA/collagen/PLA scaffold. Stem Cells Int. 2016;2016:1-13. doi: 10.1155/2016/8741641
- Li Y, Liao C, Tjong SC. Synthetic biodegradable aliphatic polyester nanocomposites reinforced with nanohydroxyapatite and/or graphene oxide for bone tissue engineering applications. Nanomaterials. 2019;9(4):1-56. doi: 10.3390/nano9040590
- Aboudzadeh N, Khavandi A, Javadpour J, Shokrgozar MA, Imani M. Effect of Dioxane and N-Methyl-2-pyrrolidone as a Solvent on Biocompatibility and Degradation Performance of PLGA/nHA Scaffolds. Iran Biomed J. 2021; 25(6): 408-416. doi: 10.52547/ibj.25.6.408
- Galindo TG, Chai Y, Tagaya M. Hydroxyapatite nanoparticle coating on polymer for constructing effective biointeractive interfaces. J Nanomater. 2019;2019:1-23. doi: 10.1155/2019/6495239
- Friuli M, Nitti P, Madaghiele M, Demitri C. A possible method to avoid skin effect in polymeric scaffold produced through thermally induced phase separation. Results Eng. 2021;12:1-3. doi: 10.1016/j.rineng.2021.100282
- Raeisdasteh Hokmabad V, Davaran S, Ramazani A, Salehi R. Design and fabrication of porous biodegradable scaffolds: a strategy for tissue engineering. J Biomater Sci Polym Ed. 2017;28(16):1797-1825. doi: 10.1080/09205063.2017.1354674
- Tan HL, Dahlan NA, Janarthanan P. Nanotechnology in Tissue Engineering and Implant Development. In Nanotechnology in Medicine 2021 (pp. 241-265). Springer, Cham. doi: 10.1007/978-3-030-61021-0_13
- Stocco TD, Bassous NJ, Zhao S, Granato AE, Webster TJ, Lobo AO. Nanofibrous scaffolds for biomedical applications. Nanoscale. 2018;10(26):12228-12255. doi: 10.1039/C8NR02002G
- Díaz E, Molpeceres AL, Sandonis I, Puerto I. PLLA/nHA composite films and scaffolds for medical implants: In vitro degradation, thermal and mechanical properties. J Inorg Organomet Polym. 2019;29(1):121-131. doi: 10.1007/s10904-018-0972-y
- Li L, Zhou C. Tissue engineering scaffolds derived from chitosan. Curr Org Chem. 2018;22(7):708-719. doi: 10.2174/1385272821666171019144752
- Wang W, Miao Y, Zhou X, Nie W, Chen L, Liu D, et al. Local delivery of BMP-2 from poly (lactic-co-glycolic acid) microspheres incorporated into porous nanofibrous scaffold for bone tissue regeneration. J Biomed Nanotechnol. 2017;13(11):1446-1456. doi: /10.1166/jbn.2017.2445
- Hikmawati D, Widiyanti P, Amrillah T, Nia W, Firdania IT, Abdullah CA. Study of mechanical and thermal properties in nano-hydroxyapatite/chitosan/carboxymethyl cellulose nanocomposite-based scaffold for bone tissue engineering: the roles of carboxymethyl cellulose. Appl Sci. 2020;10(19):1-11. doi: 10.3390/app10196970
- Tan GZ, Zhou Y. Electrospinning of biomimetic fibrous scaffolds for tissue engineering: a review. Int J Polym Mater Polym Biomater. 2020;69(15):947-960. doi: 10.1080/00914037.2019.1636248
- Collins MN, Ren G, Young K, Pina S, Reis RL, Oliveira JM. Scaffold fabrication technologies and structure/function properties in bone tissue engineering. Adv Funct Mater. 2021;31(21):1-22. doi: 10.1002/adfm.202010609
- Das A, Balakrishnan N, Joyner JD, Medhavi N, Manaf O, Jabeen Fatima MJ, et al. Electrospinning: the state of art technique for the production of nanofibers and nanofibrous membranes for advanced engineering applications. Electrospin Adv Energy Storage Appl. 2021:23-71. doi: 10.1007/978-981-15-8844-0_2
- Mortimer CJ, Wright CJ. The fabrication of iron oxide nanoparticle‐nanofiber composites by electrospinning and their applications in tissue engineering. Biotechnol J. 2017;12(7):1-10. doi: 10.1002/biot.201600693
- Mabrouk M, Beherei HH, Das DB. Recent progress in the fabrication techniques of 3D scaffolds for tissue engineering. Mater Sci Eng C. 2020;110:1-12. doi: 10.1016/j.msec.2020.110716
- Ifegwu OC, Anyakora C. The place of electrospinning in separation science and biomedical engineering. Electrospinning Method Used to Create Functional Nanocomposites Films; Tanski, TA, Jarka, P., Matysiak, W., Eds. 2018:p.17.
- Keshvardoostchokami M, Majidi SS, Huo P, Ramachandran R, Chen M, Liu B. Electrospun nanofibers of natural and synthetic polymers as artificial extracellular matrix for tissue engineering. Nanomaterials. 2020;11(1):1-23. doi: 10.3390/nano11010021
- Kalantari K, Afifi AM, Jahangirian H, Webster TJ. Biomedical applications of chitosan electrospun nanofibers as a green polymer–Review. Carbohydr Polym. 2019;207:588-600. doi: 10.1016/j.carbpol.2018.12.011
- Wei K, Li Y, Kim KO, Nakagawa Y, Kim BS, Abe K, et al. Fabrication of nano‐hydroxyapatite on electrospun silk fibroin nanofiber and their effects in osteoblastic behavior. J Biomed Mater Res A. 2011;97(3):272-280. doi: 10.1002/jbm.a.33054
- Kashte S, Jaiswal AK, Kadam S. Artificial bone via bone tissue engineering: current scenario and challenges. Tissue Eng Regen Med. 2017;14(1):1-14. doi: 10.1007/s13770-016-0001-6
- Lombardo D, Calandra P, Pasqua L, Magazù S. Self-assembly of organic nanomaterials and biomaterials: the bottom-up approach for functional nanostructures formation and advanced applications. Materials. 2020;13(5):1-42. doi: 10.3390/ma13051048
- Mi HY, Jing X, Napiwocki BN, Li ZT, Turng LS, Huang HX. Fabrication of fibrous silica sponges by self-assembly electrospinning and their application in tissue engineering for three-dimensional tissue regeneration. Chem Eng J. 2018;331:652-662. doi: 10.1016/j.cej.2017.09.020
- Nie W, Peng C, Zhou X, Chen L, Wang W, Zhang Y, et al. Three-dimensional porous scaffold by self-assembly of reduced graphene oxide and nano-hydroxyapatite composites for bone tissue engineering. Carbon. 2017;116:325-337. doi: 10.1016/j.carbon.2017.02.013
- Nahanmoghadam A, Asemani M, Goodarzi V, Ebrahimi‐Barough S. Design and fabrication of bone tissue scaffolds based on PCL/PHBV containing hydroxyapatite nanoparticles: dual‐leaching technique. J Biomed Mater Res A. 2021;109(6):981-993. doi: 10.1002/jbm.a.37087
- Olmo C, Franco L, Vidal A, del Valle LJ, Puiggalí J. Ultrasound micromolding of porous polylactide/hydroxyapatite scaffolds. Express Polym Lett. 2021;15(5):389-403. doi:10.3144/expresspolymlett.2021.34
- Soundarya SP, Menon AH, Chandran SV, Selvamurugan N. Bone tissue engineering: Scaffold preparation using chitosan and other biomaterials with different design and fabrication techniques. Int J Biol Macromol. 2018;119:1228-1239. doi: 10.1016/j.ijbiomac.2018.08.056
- Eltom A, Zhong G, Muhammad A. Scaffold techniques and designs in tissue engineering functions and purposes: a review. Adv Mater Sci Eng. 2019;2019:1-13. doi: 10.1155/2019/3429527
- Baino F, Fiume E, Magnaterra G, Verné E. Additive Manufacturing of Bioceramic Scaffolds for Bone Tissue Regeneration with Emphasis on Stereolithographic Processing. In3D printable Gel-inks for Tissue Engineering 2021 (pp. 297-331). Springer, Singapore. doi: 10.1007/978-981-16-4667-6_9
- Neto AS, Ferreira JM. Synthetic and marine-derived porous scaffolds for bone tissue engineering. Materials. 2018;11(9):1-40. doi: 10.3390/ma11091702
- Wang X, Zhang G, Qi F, Cheng Y, Lu X, Wang L, et al. Enhanced bone regeneration using an insulin-loaded nano-hydroxyapatite/collagen/PLGA composite scaffold. Int J Nanomedicine. 2018; 13: 117-127. doi: 10.2147/IJN.S150818
- Jang SJ, Kim SE, Han TS, Son JS, Kang SS, Choi SH. Bone regeneration of hydroxyapatite with granular form or porous scaffold in canine alveolar sockets. In Vivo. 2017;31(3):335-341. doi: 10.21873/invivo.11064
- Wu S, Xiao Z, Song J, Li M, Li W. Evaluation of BMP-2 enhances the osteoblast differentiation of human amnion mesenchymal stem cells seeded on nano-hydroxyapatite/collagen/poly (l-lactide). Int J Mol Sci. 2018;19(8):1-16. doi: 10.3390/ijms19082171
- Yan X, Song G, Wang X, Zhao Y, Chen Y. The preparation and medical applications of chitosan microspheres. Curr Org Chem. 2018;22(7):720-733. doi: 10.2174/1385272821666170830112633
- Sunandhakumari VJ, Vidhyadharan AK, Alim A, Kumar D, Ravindran J, Krishna A, et al. Fabrication and in vitro characterization of bioactive glass/nano hydroxyapatite reinforced electrospun poly (ε-caprolactone) composite membranes for guided tissue regeneration. Bioengineering. 2018;5(3):1-20. doi: 10.3390/bioengineering5030054
- Freeman FE, Pitacco P, Van Dommelen LH, Nulty J, Browe DC, Shin JY, et al. Development of a 3D bioprinted scaffold with spatio-temporally defined patterns of BMP-2 and VEGF for the regeneration of large bone defects. Bio Protoc. 2021;11(21):1-7. doi: 10.21769/BioProtoc.4219
This is an open-access article distributed under the terms of the CreativeCommons Attribution License (CC BY) 4.0 https://creativecommons.org/licenses/by/4.0/