By Mehwish Sajjad¹, Shaheen Kouser², Yusra², Fatima Arshad²
AFFILIATIONS:
- Department of Pathology (Microbiology), Dow University of Health Sciences.
- Department of Pathology (Haematology), Dow University of Health Sciences, Karachi, Pakistan.
ABSTRACT
Hepatitis B virus (HBV) can evolve under selection pressure exerted by drugs and host immunity, resulting in the accumulation of escape mutations that can affect the drug or the immune activity. Hepatitis B virus polymerase lacks proofreading ability and is, therefore, error prone which results in the incorporation of mismatch bases causes mutations in the Hepatitis B virus genome. Factors, which might contribute to a high Hepatitis B virus mutation rate, are drugs, host immune system and co infection. Hepatitis Delta Virus (HDV) co-infection is also known to exert selection pressure on Hepatitis B virus. This further leads to selective amplification of certain mutations, especially in genes that are required for hepatitis delta virus pathogenesis, such as Hepatitis B virus surface antigen. Some of these mutations affected the generation of proteasomal sites, binding of Hepatitis B virus surface antigen epitopes to major histocompatibility I and II ligands, and subsequent generation of B- and T-cell epitopes. Information and data from 2010 to 2020 were retrieved from search engines like PubMed, Medline, Google Scholar and Web of Science through original papers and reviews. This review focuses on mutations that the Hepatitis B virus selectively amplifies in presence of Hepatitis D virus co-infection and its effect on epitope generation.
Keywords: Hepatitis B Virus; Hepatitis D Virus; Co Infection; Epitopes; Hepatitis B Virus Surface Antigen.
Hepatitis B virus (HBV) is associated with hepatitis, cirrhosis and hepatocellular carcinoma, which is the fifth prominent cause of death worldwide1. Hepatitis B virus is an enveloped virus with double stranded Deoxyribonucleic acid (DNA) of 3.2 kilo base pairs. HBV polymerase and surface genes (HBsAg) play a crucial role in HBV genetic variability, drug resistance and antigen modification2. Hepatitis B surface antigen (HBsAg) has an important role in immune response and epitope formation, in occult blood infection, as well as a detection marker, as a receptor, and as a mediator of carcinogenesis. Furthermore, HBsAg has a major hydrophilic region that lies between amino acids 100-167, which has an ‘a-determinant’ that contributes to neutralizing antibody response. Mutations in ‘a’ determinant region cause conformational changes that can affect HBsAg antigenicity and processing and presentation of B and T cell epitopes, resulting in escape from vaccine-induced immunity and anti-HBV immunoglobulin therapy leading to failure in viral clearance.
HBV is found to co-infect with, as Hepatitis D virus (HDV) is a defective virus that requires HBV surface antigen for its replication and assembly. Therefore, HBV and HDV co-infection and super-infection leads to more severe fulminant hepatitis4. HBV amplifies those mutations in HDV presence that enables HBV to respond to the inhibitory effects of HDV replication and also the immunological recognition of HBsAg epitopes. This review mainly focuses on the identification of certain mutations in HBsAg in HBV mono and HBV-HDV co infected groups and the impact of these mutations on proteasomal degradation and CD4, CTL and B cell epitope repertoire.
Hepatitis B virus has high mortality and is one of the major causes of occult blood infection. Hepatitis D virus is a defective virus that needs Hepatitis B virus for its replication. Both causes more life-threatening fulminant hepatitis. This co infection results in selective amplification of these mutations at certain strategic locations might not only enable HBV to counteract the inhibitory effects of HDV on HBV replication but also facilitate its survival by escaping the immune response. In occult blood infection diagnosis and vaccination response of the Hepatitis B Virus is lost as diagnostic kits and vaccinations were designed against wild type Hepatitis B Virus.
Hepatitis B Virus Structure
HBV is an enveloped virus with icosahedral symmetry. Hepatitis B virus has a 3.2 kbp, partially double stranded DNA genome, enclosed in nucleocapsid core5. HBV genome shows diversity due to overlapping open reading frames. The genome of HBV is organized in such a way that its DNA is extremely compact. It leads to highly evolved organization of regulatory and promoter region results in the usage of each nucleotide in the genome. HBV genome is comprised of four overlapping open reading frame (ORF) that codes for seven different proteins as shown in Figure 1A.
The polymerase is 832 amino acid long protein, coded by the largest ORF and has four domains: terminal domain has N terminus, spacer domain, RT domain which houses the polymerase activity, RNase H domain at C terminal portion6,7. The Pre-core/core ORF codes for the translation of Pre-core and core protein. Core protein helps in the formation of a nucleocapsid core that encloses the polymerase. Pre-core protein, also known as Hepatitis B virus e antigen (HBeAg), is used for the assessment of viral proliferation, disease progression and severity and drug response. X protein of HBV is encoded by the smallest ORF8, which has a significant role in cell proliferation, the prognosis of infection and drug response. Several studies have implicated HBV X proteins in hepatocellular carcinoma9. The surface ORF is the second largest ORF that codes for the large, middle and small surface antigen proteins.
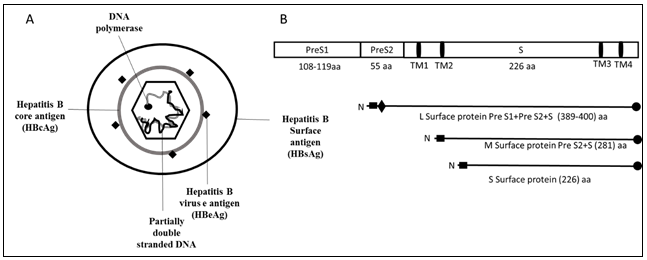
Figure 1: Structure of HBV and genomic organization of HBsAg. A) HBV is an enveloped virus surrounded by nucleocapsid core. Nucleocapsid core has partially double stranded DNA, has polymerase at one end. B) Organization of surface protein. HBV surface antigen has three proteins: large surface antigen has Pre S1, Pre S2 and S domain, middle surface antigen has Pre S2 and S domain and small surface antigen has only S domain.
Hepatitis B Virus Surface Antigen and Mutations
Hepatitis B virus surface antigen (HBsAg) is an envelope protein. There are three forms of envelope proteins i.e., Large HBsAg, Medium HBsAg and Small HBsAg. All three forms get transcribed from the same single ORF, which has three, in frame initiation sites. These proteins are the translational product of two sub-genomic mRNAs. A Sub-genomic RNA of 2.4kb gives rise to large surface protein, whereas another 2.1kb gets translated into middle and small surface proteins10. Divergence in the manifestation of these surface proteins is due to sub-genomic RNAs that are regulated by preS1 and preS2/S promoters11 that lead to variable initiating amino-terminal. Small surface protein has 226 amino acids and only has S domain. Middle surface protein has S domain and additional 55 amino acids at N terminal in the form of preS2. Large surface protein has 400 amino acids and has preS1, preS2 and S domain with an addition of 109 amino acids at the amino terminus (N-terminus) in the form of preS1 as shown in Figure 1B. These proteins help in the formation of HBV envelop, entry of virus by binding to sodium taurocholate co-transporting polypeptide receptors on hepatocytes, viral replication, formation and secretion12-14.
From the host perspective, HBsAg is also very critical as a mutation in this region can alter the virus infectivity, antigenicity, failure in the detection and immune response15,16. Small domain of HBsAg has a-determinant region, comprising of amino acid 124-147 in the major hydrophilic region of amino acids located between 100-169 amino acid. This region has a great significance as it resides dominant epitopes which are the target of neutralizing B cell response17. Mutation in HBsAg led to an occult blood infection, diagnostic failure, immune evasion, vaccine escape and drug resistance, all of these are the hallmark of hepatitis B virus surface antigen mutation18. It has been shown in a study that HBsAg mutations C124R, C124Y, K141E and D144A can reduce the immunoassay detection, whereas mutations G119R, S136P, D144A, I126S, Q129R and G144R result in reducing S protein and virion secretion. Mutations in HBsAg at G145R result in vaccine escape, diagnostic failure, and reduced binding with monoclonal anti-HBs. Mutation K122I in HBsAg affects the interaction of HBV with anti-HBs (anti Hepatitis B virus surface) antibodies. Furthermore, mutations Y100S, G119R, T126I, G145R, T140I, M133T and Y134C have been associated with HBV occult blood infection19-22. Additionally, various vaccine escape mutations (K141E, M133L, T116N, P142S, and G145R) are also observed in HBsAg.
Hepatitis B Virus Pathogenesis and Immune Response
HBV is a hepatotropic, noncytopathic virus that causes a spectrum of liver diseases. Chronic progression of HBV infection its persistence and clinical consequences depend on both host and viral factors. Direct interaction of HBV and host immunity causes T cells damage to the hepatocytes. A robust immune reaction occurs in acute HBV infection that results in viral clearance and self-limited liver inflammation; whereas in chronic HBV infection, the host is unable to mount efficient innate and adaptive immune response23,24. HBV evades innate immunity at various steps, such as it inhibits the activation of Toll-like receptors (TLRs), natural killer cells and production of various cytokines and interferon, which are essential to control HBV infection25-27. HBV also evades innate immune response by producing various viral proteins that engage various components of innate immunity28.
Various components of adaptive immunity against HBV include HBV specific antibodies, B cells, TH cells (Helper T cells) and TC cells (Cytotoxic T cells)29. Numerous studies have shown that there is a great increase in B cells, IgM, IgG and plasma cells in chronic HBV infection30,31. Other studies have reported contradictory findings, for example, few have reported a decrease in the level of anti-HBs antibody and CD80 specific B cell in chronic HBV infection, while others have reported no difference in their levels24, 32, 33. A high level of anti-HBs antibodies indicate viral clearance, immunity after vaccination and are also used as diagnostic tool24,34. The role of cell mediated immunity; such as one mediated by CD4 T lymphocytes and CD8 T lymphocytes have a major role in HBV eradication. Various reports suggest that early CD4 T cells are dominant for the initiation of functional HBV specific CD8 T cell response. HBV specific CD4 T cells start appearing in circulation simultaneously with CD8 T cells after a month of infection in response to active viral replication. CD4 T cells mostly recognized epitopes of the core protein. Different stages of chronic HBV infection show variable counts of T cells, where low CD4 count is observed in the inactive carrier stage. In the chronic stage, HBV-specific T cells are unable to proliferate and produce cytokines34. In acute HBV infection, CD4 and CD8 actively secrete interferon with extensive epitope recognition as compared to chronic HBV infection. Prolonged and continuous secretion of HBV surface antigen in large quantity altersthe functions of B and T cells.
Human Leukocyte Antigen (HLA) and HBV Infection
The Human Leukocyte Antigen (HLA) system is the highly polymorphic system, located on chromosome 6, and has a great impact on the course of HBV infection35. HLA-I and HLA-II present peptides on the infected cell surface, where they are recognized by the CD8 and CD4 T lymphocytes, respectively. High polymorphism of HLA influences HBV persistence and clearance, therapeutic regimen and vaccine efficacy. It has been shown in many studies that MHC class II DRB1*1302, DRB1*1502, DQB1*0302, DQB1*0609, DR*04 and DR*13 is associated with HBV clearance. MHC class 1 allele HLA -A0206, -B35, -B18, -B40, -A03 and -B18 are associated with chronicity in different global populations. HLA -A1, -A2, -A10, -A11, -B7, -B8, -B15, -B40 and -B54 are associated with non-responsiveness to HBV vaccine in various world populations. HLA -B8, -B35 and -B40 are associated with chronic delta infection in a Russian population. It has also been reported that HLA-DR13 is associated with vertical transmission of HBV. Hence, diversity in HLAs has various impacts and associations with the clinical course of HBV in different ethnicities of the world36,37.
Effect of HBV Surface Antigen Mutations
HBV infected hepatocytes are mostly degraded by proteasome into epitopes, which are then presented to the cell surface in conjugation with MHC-1 molecules that are then recognized by the cytotoxic T lymphocytes (CTL). Multiple studies have shown that proteasome degrades HBsAg into short length peptides. Mutation in HBsAg amino acids leads to the generation of those viral peptides that disrupt proteasomal degradation process and abrogates the immune response against the epitope. Mutations in HBsAg can also lead to altered HLA binding, which affects downstream response by CD8, CD4 and B cell epitope, and is the known mechanism of immune escape. The most important and first documented mutation seen in the ‘a-determinant’ region is G145R, which is a vaccine escape mutation. Amino acid mutations in a region spanning amino acids 160-207 affect B cell epitope38. A study has shown that HLA-DRB1*0101 restricted CD4 epitopes assisted in the generation of CD8 T cell response. It has been demonstrated in a study that vaccination helps in the induction of CD4 T cell response against HBV surface antigen. Two novel HLA-DR1 restricted epitopes S109-134 and S200-214 were demonstrated in a study, which is helpful in monitoring the efficacy of CD4 T cell response in vaccinated individuals. It has been reported in a study that amino acid sequence in HBsAg from 298-321 is recognized by HBV anti-surface antibodies, whereas the amino acids 298 to 311 and 313 to 321 are recognized by CD4 T cells. A study identified four epitopes in HBsAg from amino acids 21-40, 136-155, 156-175 and 211-226 that can produce helper T cell response presented by HLA-class II alleles.
HBV Co-infection
Co-infections in viruses influence the disease pattern that occurs relative to that with mono- infection. Presence of an extra virus also hinder with the targeted isolation of a known virus. Viral interference is a phenomenon in which a virus competitively suppresses replication of other co-infecting viruses, which is the most common outcome of viral co-infections. Co-infections alter disease severity and epidemiology by modulating its virulence and cell death. Immune responses to primary virus infection also regulate immune responses to subsequent secondary viral infections. HBV can be found co-infected with HIV, HCV and HDV39-41. Co-infections were mostly observed when the viruses share similar transmission routes. Like HIV, HCV also transmitted vertically through blood transfusion and by organ transplantation. Moreover, all hepatitis viruses target similar organ, like HCV and HDV both affect the liver. It assumes that these viruses might share receptors. In this review, we focused on HBV-HDV co-infection as they both are a hepatotropic virus, share similar modes of transmission, affect the same organ. HDV needs HBsAg for its assembly and completion of its life cycle. In HBV-HDV co-infection phenomenon of viral interference is observed as HDV is a satellite virus and needs HBsAg for its assembly, packaging and completion of its life cycle.
Hepatitis D Virus
Hepatitis Delta virus (HDV) was noticed and discovered by Dr. Mario Rizzetto and colleagues in late 1977, during staining of hepatocytes for HBV. HDV is known as a satellite and defective virus as it needs HBsAg to complete its life cycle and propagation42. Among 350 million HBV carriers, there are 15 to 20 million (5% of HBV carriers), which are dually infected with HDV globally43. Among HBV carriers the prevalence of HDV in Pakistan is approximately 16.6%. HBV-HDV co-infection leads to liver cirrhosis, fulminant hepatitis and hepatocellular carcinoma44. HDV has a single-stranded negative sense genome of 1.7kb45. HDV is a unique virus as it contains circular RNA HDV genome has protein coding region with only one open reading frame that encodes delta antigen and viroid like region have ribozyme activity. Delta antigen exists in two isomeric forms, large delta antigen having 214 residues, whereas small delta antigen has 195 residues. A ribonucleoprotein (RNP) complex is formed when delta antigen is associated with genomic RNA. This ribonucleoprotein complex (RNP) is enveloped by endoplasmic derived HBsAg having large, middle and small surface protein46. HDV can be contracted in two ways as shown in Table 1. HDV occurs concurrently with HBV leads to co-infection like superinfection in which chronic HBV carrier interacts with new HDV infection. This chronic HDV infection deteriorates the routine functions of liver and damages it.
Table 1: The difference between co-infection and superinfection.
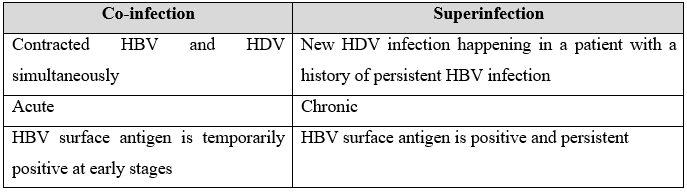
The hepatitis D virus is the dominant virus in co-infection and superinfection with HBV since HDV represses hepatitis B virus replication. Evidence shows 70 to 90% of co-infected persons are negative for HBeAg and exhibit low levels of HBV viral load. One possible mechanism through which HBV replication is suppressed by HDV is through small p24 and large p27 proteins encoded by HDV. These proteins inhibit enhancer regions of HBV. Additionally, the p27 protein of HDV stimulates interferon α inducible MxA-gene, which also plays a role in suppressing HBV replication46,47.
Role of HBsAg and HBsAg Mutation in HBV-HDV Co-infection
Certain studies have shown that HDV influences the functional properties of HBV46. HDV restricts HBV replication by encoding p24 and p27 proteins that impede enhancer activity of HBV. Moreover, p27 from HDV triggers interferon α inducible MxA-gene production that stops HBV replication. Codons at position 196 of HBsAg assist HDV in the packaging48. It is illustrated in many studies that mutations in HBsAg, mainly in residues 163 to 224 of C terminal might result in a severe reduction of HDV assembly. It is documented in few studies that antiviral drugs also induce mutation that also found in this domain. It is shown in the study that mutations at the C terminus positions 204 and 206 of HBsAg can interrupt the interaction between HBsAg and large hepatitis delta antigens (L-HDVAg) that leads to improper envelope formation. HDV co-infection can exert selection pressure on HBsAg leading to its evolution48.
Several studies have previously shown that interference between the two viruses in HBV-HDV co infection setting effect their co-evolution, allowing selective amplification of certain mutations in HBsAg that are specific to either HBV mono infection or HBV and HDV co-infected sequences49. Amplification of mutations in HBV in the backdrop of HDV might be a mechanism by which HBV counteracts the inhibitory effects of HDV on its replication. However, it has not been explored in depth how HBsAg mutations affect the immunological recognition of HBsAg epitopes50. Therefore, in this review, we have explored how HBsAg mutations occurring in the backdrop of HDV which further affect B and T cell epitope processing.
HBV selectively amplifies certain mutations in the co-infection. Some of the mutations are strategically localized in regions that are vital for HDV replication or activity of immune cells (including clearance of HBV by the immune cells). Selective amplification of these mutations might not only enable HBV to counteract the inhibitory effects of HDV on HBV replication but also facilitate its survival by escaping the immune response. However, further studies are required to confirm these observations. Even so, this analysis might help in tailor-designing vaccines that are effective in HBV mono- or HBV-HDV co-infected cases.
The author is thankful to all colleagues and friends for continuous support and assistance.
The authors declare no conflict of interest.
The corresponding author had collected all the data and written this review. The remaining co-authors crosschecked the manuscript, helped in compiling the data and participated in discussions for presenting the review article for publication.
- Gish RG, Given BD, Lai CL, Locarnini SA, Lau JY, Lewis DL, et al. Chronic hepatitis B: Virology, natural history, current management and a glimpse at future opportunities. Antiviral Res. 2015;121:47-58.
- Verbinnen T, Hodari M, Talloen W, Berke JM, Blue D, Yogaratnam J, et al. Virology analysis of chronic hepatitis B virus-infected patients treated for 28 days with JNJ-56136379 monotherapy. J Viral Hepat. 2020;27(11):1127-1137.
- Alvarado-Mora MV, Locarnini S, Rizzetto M, Pinho JR. An update on HDV: virology, pathogenesis and treatment. Antivir Ther. 2013;18(3 Pt B):541-548.
- Ghamari S, Alavian SM, Rizzetto M, Olivero A, Smedile A, Khedive A, et al. Prevalence of hepatitis delta virus (HDV) infection in chronic hepatitis B patients with unusual clinical pictures. Hepat Mon. 2013;13(8):1-8.
- Li X, Zhao J, Yuan Q, Xia N. Detection of HBV covalently closed circular DNA. Viruses. 2017;9(6)1-19.
- Unchwaniwala N, Sherer NM, Loeb DD. Hepatitis B virus polymerase localizes to the mitochondria, and its terminal protein domain contains the mitochondrial targeting signal. J Virol. 2016;90(19):8705-8719.
- Clark DN, Hu J. Unveiling the roles of HBV polymerase for new antiviral strategies. Future Virol. 2015;10(3):283-295.
- Ali A, Abdel-Hafiz H, Suhail M, Al-Mars A, Zakaria MK, Fatima K, et al. Hepatitis B virus, HBx mutants and their role in hepatocellular carcinoma. World J Gastroenterol. 2014;20(30):10238-10248.
- Zhang Y, Liu H, Yi R, Yan T, He Y, Zhao Y, et al. Hepatitis B virus whole-X and X protein play distinct roles in HBV-related hepatocellular carcinoma progression. J Exp Clin Cancer Res. 2016;35(1):1-10.
- Glebe D, Bremer CM. The molecular virology of hepatitis B virus. Semin Liver Dis. 2013;33(2):103-112.
- Chen J, Liu Y, Zhao J, Xu Z, Chen R, Si L, et al. Characterization of novel hepatitis B virus preS/S-Gene mutations in a patient with occult hepatitis B virus infection. PLoS One. 2016;11(5):1-14.
- Yan H, Zhong G, Xu G, He W, Jing Z, Gao Z, et al. Sodium taurocholate cotransporting polypeptide is a functional receptor for human hepatitis B and D virus. elife. 2012;1:1-28.
- Pollicino T, Amaddeo G, Restuccia A, Raffa G, Alibrandi A, Cutroneo G, et al. Impact of hepatitis B virus (HBV) preS/S genomic variability on HBV surface antigen and HBV DNA serum levels. Hepatol. 2012;56(2):434-443.
- Toita R, Kawano T, Kang JH, Murata M. Applications of human hepatitis B virus preS domain in bio- and nanotechnology. World J Gastroenterol. 2015;21(24):7400-7411.
- Biswas S, Candotti D, Allain JP. Specific amino acid substitutions in the S protein prevent its excretion in vitro and may contribute to occult hepatitis B virus infection. J Virol. 2013;87(14):7882-7892.
- Powell EA, Boyce CL, Gededzha MP, Selabe SG, Mphahlele MJ, Blackard JT. Functional analysis of ‘a’ determinant mutations associated with occult HBV in HIV-positive South Africans. J GenVirol. 2016;97(7):1615-1624.
- El Hadad S, Alakilli S, Rabah S, Sabir J. Sequence analysis of sub-genotype D hepatitis B surface antigens isolated from Jeddah, Saudi Arabia. Saudi J Biol Sci. 2018;25(4):838-847.
- Kim JH, Moon HW, Ko SY, Choe WH, Kwon SY. Hepatitis B surface antigen levels at 6 months after treatment can predict the efficacy of lamivudine-adefovir combination therapy in patients with lamivudine-resistant chronic hepatitis B. Clin Mol Hepatol. 2014;20(3):274-282.
- Svicher V, Cento V, Bernassola M, Neumann-Fraune M, Van Hemert F, Chen M, et al. Novel HBsAg markers tightly correlate with occult HBV infection and strongly affect HBsAg detection. Antiviral Res. 2012;93(1):86-93.
- Huang CH, Yuan Q, Chen PJ, Zhang YL, Chen CR, Zheng QB, et al. Influence of mutations in hepatitis B virus surface protein on viral antigenicity and phenotype in occult HBV strains from blood donors. J Hepatol. 2012;57(4):720-729.
- Huang X, Qin Y, Li W, Shi Q, Xue Y, Li J, et al. Molecular analysis of the hepatitis B virus presurface and surface gene in patients from eastern China with occult hepatitis B. J Med Virol. 2013;85(6):979-986.
- Lazarevic I. Clinical implications of hepatitis B virus mutations: recent advances. World J Gastroenterol. 2014;20(24):7653-7664.
- Liaw YF, Chu CM. Immune Tolerance phase of chronic hepatitis B. Gastroenterol. 2017;152(5):1245-1246.
- Wang L, Wang K, Zou ZQ. Crosstalk between innate and adaptive immunity in hepatitis B virus infection. World J Hepatol. 2015;7(30):2980-2991.
- Ma Z, Zhang E, Yang D, Lu M. Contribution of Toll-like receptors to the control of hepatitis B virus infection by initiating antiviral innate responses and promoting specific adaptive immune responses. Cell Mol Immunol. 2015;12(3):273-282.
- Pei RJ, Chen XW, Lu MJ. Control of hepatitis B virus replication by interferons and Toll-like receptor signaling pathways. World J Gastroenterol. 2014;20(33):11618-11629.
- Yang A, Hu D, You H, Wang B. [Natural killer cells play an important role in hepatitis C infection]. Chin J Hepatol. 2014;22(10):798-800.
- Revill P, Yuan Z. New insights into how HBV manipulates the innate immune response to establish acute and persistent infection. Antivir Ther. 2013;18(1):1-15.
- Boeijen LL, Hoogeveen RC, Boonstra A, Lauer GM. Hepatitis B virus infection and the immune response: The big questions. Best Pract Res Clin Gastroenterol. 2017;31(3):265-272.
- Oliviero B, Cerino A, Varchetta S, Paudice E, Pai S, Ludovisi S, et al. Enhanced B-cell differentiation and reduced proliferative capacity in chronic hepatitis C and chronic hepatitis B virus infections. J Hepatol. 2011;55(1):53-60.
- Mohamadkhani A, Naderi E, Sotoudeh M, Katoonizadeh A, Montazeri G, Poustchi H. Clinical feature of intrahepatic B-lymphocytes in chronic hepatitis B. Int J Inflam. 2014;2014:1-6.
- Xu X, Shang Q, Chen X, Nie W, Zou Z, Huang A, et al. Reversal of B-cell hyperactivation and functional impairment is associated with HBsAg seroconversion in chronic hepatitis B patients. Cell Mol Immunol. 2015;12(3):309-316.
- Wongjitrat C, Sukwit S, Chuenchitra T, Seangjaruk P, Rojanasang P, Romputtan P, et al. CTLA-4 and its ligands on the surface of T- and B-lymphocyte subsets in chronic hepatitis B virus infection. J Med Assoc Thai. 2013;96 (Suppl 1):S54-S59.
- Vanwolleghem T, Hou J, van Oord G, Andeweg AC, Osterhaus AD, Pas SD, et al. Re-evaluation of hepatitis B virus clinical phases by systems biology identifies unappreciated roles for the innate immune response and B cells. Hepatol. 2015;62(1):87-100.
- Akcay IM, Katrinli S, Ozdil K, Doganay GD, Doganay L. Host genetic factors affecting hepatitis B infection outcomes: Insights from genome-wide association studies. World J Gastroenterol. 2018;24(30):3347-3360.
- Matsuura K, Isogawa M, Tanaka Y. Host genetic variants influencing the clinical course of hepatitis B virus infection. J Med Virol. 2016;88(3):371-379.
- Wang L, Zou ZQ, Wang K. Clinical relevance of HLA gene variants in HBV infection. J Immunol Res. 2016;2016:1-8.
- Lin YM, Jow GM, Mu SC, Chen BF. Naturally occurring hepatitis B virus B-cell and T-cell epitope mutants in hepatitis B vaccinated children. Sci World J. 2013;2013:1-8.
- Hu J, Liu K, Luo J. HIV-HBV and HIV-HCV coinfection and liver cancer development. Cancer Treat Res. 2019;177:231-250.
- Gong DY, Chen EQ, Huang FJ, Leng XH, Cheng X, Tang H. Role and functional domain of hepatitis B virus X protein in regulating HBV transcription and replication in vitro and in vivo. Viruses. 2013;5(5):1261-1271.
- Sanou AM, Benkirane K, Tinto B, Cisse A, Sagna T, Ilboudo AK, et al. Prevalence of hepatitis B virus and hepatitis D virus coinfection in Western Burkina Faso and molecular characterization of the detected virus strains. Int J Infect Dis. 2018;70:15-19.
- Rizzetto M. The delta agent. Hepatol. 1983;3(5):729-737.
- Scarponi CF, Silva RD, Souza Filho JA, Guerra MR, Pedrosa MA, Mol MP. Hepatitis delta prevalence in South America: a systematic review and meta-analysis. Rev Soc Bras Med Trop. 2019;52:1-18.
- Huang CR, Lo SJ. Hepatitis D virus infection, replication and cross-talk with the hepatitis B virus. World J Gastroenterol. 2014;20(40):14589-14597.
- Cunha C, Tavanez JP, Gudima S. Hepatitis delta virus: A fascinating and neglected pathogen. World J Virol. 2015;4(4):313-322.
- Lempp FA, Ni Y, Urban S. Hepatitis delta virus: insights into a peculiar pathogen and novel treatment options. Nat Rev Gastroenterol Hepatol. 2016;13(10):580-589.
- Freitas N, Abe K, Cunha C, Menne S, Gudima SO. Support of the infectivity of hepatitis delta virus particles by the envelope proteins of different genotypes of hepatitis B virus. J Virol. 2014;88(11):6255-6267.
- Colagrossi L, Salpini R, Scutari R, Carioti L, Battisti A, Piermatteo L, et al. HDV Can constrain HBV genetic evolution in HBsAg: Implications for the identification of innovative pharmacological targets. Viruses. 2018;10(7):1-17.
- Baig S, Abidi SH, Azam Z, Majid S, Khan S, Khanani MR, et al. Evolution of HBV S-gene in the backdrop of HDV co-infection. J Med Virol. 2018;90(8):1328-1336.
- Shirvani-Dastgerdi E, Pourkarim MR, Herbers U, Amini-Bavil-Olyaee S, Yagmur E, Alavian SM, et al. Hepatitis delta virus facilitates the selection of hepatitis B virus mutants in vivo and functionally impacts on their replicative capacity in vitro. Clin Microbiol Infect. 2016;22(1):1-6.